A whole new world
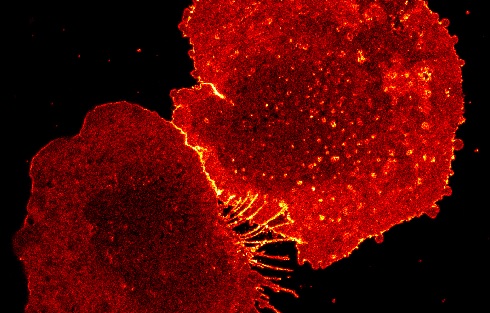
Above: The protein CD9 in an activated human macrophage, captured using super-resolution microscopy (STED) - Ashley Ambrose and Daniel M. Davis, University of Manchester
An exclusive extract from Professor Dan Davis’s new book, The Secret Body, explains how super-resolution microscopy is revealing the workings of cells in “magical and humbling” new detail
September 8th 2021
I sit in a darkened room with the temperature exquisitely controlled – there must be no flux in the environment. The machine itself fills two large tables. The main body of the microscope sits on a table that is especially bulky, because it includes a pneumatic system to isolate it from ambient vibrations in the room. To witness nature on a nanoscale, things need to be held steady on a nanoscale.
A series of metal boxes, stacked to the side of the tables, house lasers and their electronic controls, which feed light into the microscope along optical fibres. I rarely need to look down the microscope’s binocular eyepiece because what I would see is also displayed on a large computer screen in front of me. An adjacent screen shows graphics of sliders and drop-down menus to adjust the power of the lasers, the sensitivity of the light detectors, the pixel size, the speed at which the lasers scan the sample, the number of times a sample is scanned, the distance the objective lens moves to capture different depths, the pinhole size, and much more.
For anyone who hasn’t used a super-resolution microscope before, the experience is other-worldly. Going for a walk in a field, in a forest or along a trail brings us close to nature, but in a blackened room with the air hardly moving, we witness its deepest secrets.
Super-resolution images obtained in my lab have led to a new idea for treating patients with a rare genetic disease called Chediak-Higashi syndrome. Children with this syndrome are unable to fight infections that would normally be dealt with easily, and often die young. In normal circumstances, immune cells kill aberrant cells – including cancer cells or virus-infected cells – by secreting toxic enzymes into them. These enzymes are stored inside immune cells within small droplets of liquid, called lytic granules, each enclosed by a thin layer of fat molecules.
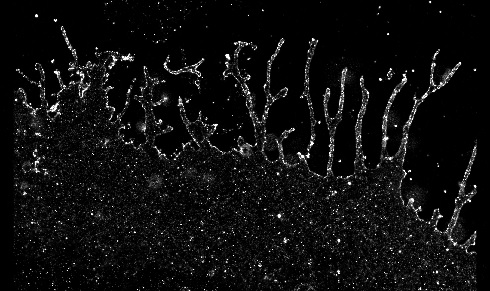
In a few minutes or so, the diseased cell visibly bulges and bubbles. Less easy to see directly, the diseased cells’ proteins and genetic material are chopped up and degraded. Remnants of the dead cell are then engulfed by another type of immune cell, where they will be broken down further and their chemical components re-used, in the same way that when we are buried, our molecular parts may be re-used by organisms in the earth.
But in children with Chediak-Higashi syndrome this process doesn’t work. Working with Polish scientist Konrad Krzewski at the US National Institute of Allergy and Infectious Diseases in Bethesda, therefore, we deliberately mutated a gene known to cause Chediak-Higashi syndrome in immune cells in a lab dish, and examined them with a super-resolution microscope.
We hoped to understand how this genetic mutation changed immune cells, to help explain why children with this syndrome are especially susceptible to certain types of infection.
We found that these genetically altered immune cells had larger-than-usual bags of toxic enzymes inside – about twice as big as normal. We discovered that they were simply too big to pass through the structural meshwork – a bit like the strings of a tennis racquet – that underlies the cell surface and gives the cell its shape, and would therefore be unable to launch an attack on diseased cells. This could indeed be part of the reason why children with this syndrome can’t deal with some types of infection very well, because their immune cells can’t easily launch an appropriate attack.
This in turn led us to think that finding a way to open up the meshwork – increase the size of the holes between the racquet strings – might restore the affected immune cell’s ability to kill diseased cells.
I knew about a drug that can do precisely this, used to treat patients with certain types of cancer, because it kept my own father alive. It is also responsible for one of the world’s worst ever medical tragedies. The use of thalidomide to help pregnant women with morning sickness led to many thousands of babies being born without fully developed limbs and with a host of other deformities. Roughly half of them subsequently died young. Nobody knows how many miscarriages were caused by the drug.
However, thalidomide was also observed to have some positive effects on various diseases, including leprosy and cancer. The US pharma company Celgene created a safer derivative of thalidomide, sold as Revlimid, by switching one oxygen atom for a nitrogen atom in its chemical structure. My father, afflicted with multiple myeloma, took this drug for many years. It’s not entirely understood how it works – thalidomide and its derivatives have many effects in the body – but one thing it does do, as we found out in my own lab, is boost the opening up of an immune cell’s structural mesh, making it easier for them to kill cancer cells.
Krzewski and I first got chatting about Chediak-Higashi syndrome at the hotel bar during a scientific meeting in Heidelberg, Germany, in September 2013: the most valuable encounters at scientific meetings are usually the informal ones. He was studying the illness directly and my lab had expertise in using super-resolution microscopy to watch immune cells kill.
Although we didn’t have any clear plan at first, it seemed like we should join forces. I had a Polish researcher in my lab at the time, Ania Oszmiana, who was also at that meeting. That she and Krzewski shared a language and culture probably helped get things going – rapport between scientists is at least as important as a good idea.
Eventually, this led us to test whether the drug my father was taking to treat his cancer might also help children with Chediak-Higashi syndrome. By the time we arrived at a clear set of experiments to do, Oszmiana had achieved her doctorate, largely based on other work using super-resolution microscopy, and she had left my lab to work in Australia. These experiments were done by an Ethiopian student in my team, Mezida Saeed.
Giving children with the syndrome the drug directly was not an option and, besides, we couldn’t then have given them a deliberate viral infection to see how they fared. Instead, we isolated immune cells from their blood and tested whether adding the thalidomide derivative would rescue their ability to kill diseased cells in a lab dish. The answer turned out to be yes, to some extent.
This is not a medical breakthrough, because we didn’t try any experiments on animals or humans, and the drug could, for example, have unwanted side-effects. But scientifically, it was a useful advance – understanding a disease and what sort of approach might work as a treatment – and all brought about by super-resolution microscopy.
In my view, there are two ways to use a super-resolution microscope. Most commonly, it is used in the way I have just described: to investigate a process we already know to be important – in this case, how toxic proteins emerge from an immune cell to kill a diseased cell – revealing crucial new detail. But the other way to use a super-resolution microscope is more akin to the way Hooke used a microscope in 1665: to explore nature, without setting out to see anything in particular.
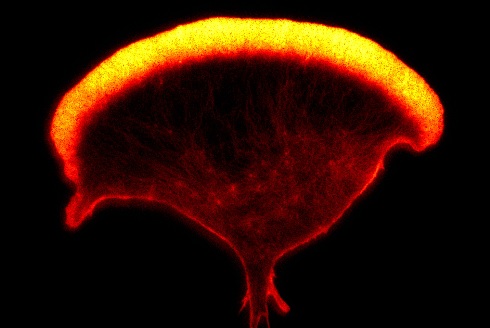
By using a super-resolution microscope simply to watch cells or combinations of cells, something entirely new might be revealed. Perhaps a new part of a cell will be discovered, or an unexpected way in which two cells interact will be witnessed. Both approaches – digging into the details of known mechanisms and open-ended exploration – are vital. But it’s the second approach that leads to the most magical feeling of discovery.
In 2016, Jennifer Lippincott-Schwartz and her team at the Howard Hughes Medical Institute used a super-resolution microscope to look at the elaborate structure inside cells where proteins are manufactured and processed, called the endoplasmic reticulum, or ER. It was thought that this structure, which fills a large part of the cell, was made up of sheets and tubes of membrane. But it turns out that this view, found in high school textbooks, wasn’t really right either.
Lippincott-Schwartz’s team revealed that the supposed sheets of membrane were in fact tubular structures too, so densely packed that when viewed under a normal microscope they looked like flat sheets of membrane. There had been nothing to suggest that this would be the case. It was an entirely unexpected discovery.
Super-resolution microscopy has set us a new challenge: understanding what this means. A dense tubular structure might increase flexibility, which could be important when the cell moves. Or it could provide greater surface area, the better to store or facilitate reactions. As yet, we do not know. Exploring cells with these new microscopes is akin to the moment you put on a new pair of prescription glasses. Details are revealed which you had no idea were there. The technology is still so new that a tremendous amount is still being discovered. In the same way that Samuel Pepys stayed up until 2am reading Hooke’s Micrographia, I relish the new views of cells described in this chapter. They reveal an intricacy to what we are, far beyond anything we might have imagined without the development of super-resolution microscopes and other tools.
These details are magical and humbling. But, personally, I find it existentially unsettling to realise how much is going on within my body without my awareness. Discoveries made with super-resolution microscopy elevate that feeling to a whole new level. The technology continues to improve. Other new microscopes are being built right now, allowing us to see more and see better. New wonders will be found that will affect our lives, not least in creating new medicines.
Professor Daniel Davis FRSB is professor of immunology at the University of Manchester. His previous books include The Beautiful Cure and The Compatibility Gene. He is currently chair of The Biologist Editorial Board.
The Secret Body: How the New Science of the Human Body Is Changing the Way We Live is available through Penguin Books.