Crazy horses
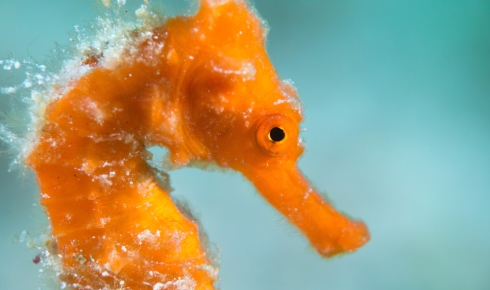
Seahorses have a unique breeding mechanism that involves males becoming pregnant. Francisco Otero-Ferrer and William V Holt FRSB explain how this unusual arrangement can help scientists conduct studies that would otherwise be impossible
The Biologist 65(3) p10
It's not surprising that many people visiting aquaria do not think seahorses are fish. After all, fishes don't generally have a horse-like head, a prehensile tail like a monkey and independently mobile eyes like a chameleon. Nor do they swim with an upright posture.
However, seahorses are indeed fishes, being close relatives of pipefishes, from which they are believed to have evolved. It's not just their unusual shape and behaviour that set seahorses apart from much of the marine world. Their reproductive biology is unique among vertebrate species, with paternal care refined to such a degree that the males actually become pregnant.
The male seahorse has an extremely complex breeding role, with just two weeks between mating and parturition (birth). Of course, in order to mate successfully, the male must first produce sperm, and thus a period of spermatogenesis, hormonally driven by androgens, is required beforehand. The androgens also stimulate the male's mating behaviour.
When it is time to mate, females deposit their eggs into a specialised brood pouch on the male's abdomen, which opens just before mating and is firmly closed afterwards. Once mating occurs, the circulating androgen concentration declines rapidly, as the male has to perform a completely different function: supporting the developing embryos.
Mating itself is accompanied by increasing circulatory progesterone concentrations within the male, which, together with increasing prolactin and glucocorticoids, support epithelial cell proliferation and secretion within the brood pouch. These are the same hormones that assist pregnancy and placental function in mammals, supporting the view that these hormones are obligatory for pregnancy, whether in males or females.
After mating, the fertilised eggs differentiate into embryos, which attach to the inner surface of the pouch walls. While these embryos largely rely on their yolk sacs for nourishment, it is becoming increasingly evident that interactions with the highly vascularised pouch walls fulfil functions that mirror those of the mammalian placenta.
The epithelial function provides mechanisms for gaseous exchange and pouch fluid osmoregulation, but experimental studies in pipefishes, where the male also possesses a brood pouch for embryonic development, have shown that embryos receive amino acids and glucose from the parent. Pouch fluid is derived from the male parent's blood serum and is known to be rich in proteins, so it is hypothesised that the brood pouch has an important role in supporting the embryos once the eggs hatch. This view is gradually gaining ground, although it is still controversial.
Mating in seahorses is preceded by lengthy courtship displays, but the process of transferring the hundreds or even thousands of eggs from the female to the male is very rapid, normally taking less than 10 seconds. How exactly those eggs are fertilised has puzzled marine biologists for many years.
The female uses her ovipositor to transfer eggs into the male's pouch, while the male releases a few thousand sperm from an externally directed sperm duct. The sperm duct is not situated next to the pouch opening, as described in some papers, but some distance from the pouch opening (about 5mm in Hippocampus kuda). But all of the sperm would be lost if ejaculated directly into the external environment, so it seems likely that the ovipositor has a role in scooping up the sperm, somehow pushing them into the pouch along with the eggs. Unfortunately, for the moment, this is a purely speculative hypothesis – the male and female genital organs are so closely apposed that it has not yet been possible to observe what is going on.
Fertilisation takes place once the eggs and sperm are within the brood pouch, together with seawater. This cannot be regarded as true external fertilisation, as it occurs inside a sealed and protected environment, although undoubtedly it does occur within seawater. Equally, it cannot be regarded as internal fertilisation, as the sperm and eggs are interacting within the equivalent of an external environment.
Fishes with reproductive strategies that involve external fertilisation (ejaculating directly into water) are well known to produce sperm in very large numbers, thus offsetting the risks associated with both sperm competition[1] and sperm wastage. However, because the mating strategy of seahorses ensures that sperm competition is almost impossible (i.e. there is no possibility that a second male could intervene during mating and introduce his sperm into his rival's pouch), there is evidently no need to produce large numbers of sperm. In fact, it has been estimated that the sperm-to-egg ratio in seahorses may be as low as 5:1.
However, to complicate matters, it seems that spermatogenesis in the male seahorse not only results in the production of rather few sperm, but that those produced come in two different sizes, with long and short tails[2,3]. It is not obvious why this adaptation is present.
We do not know whether the long or short sperm (or both) are responsible for fertilisation or whether one sperm type is actually sterile. The production of dimorphic sperm has also been observed in some pipefishes[2,4] and is known to occur in a variety of invertebrates – for example, gastropods, spiders, centipedes and insects, where the different sperm types are sometimes regarded as a way of countering sperm competition effects – that is, if males can produce infertile sperm, they can sometimes fool the females into thinking their reproductive organs are replete with sperm, thus making them less likely to mate with more males.
Several studies have shown that the fecundity of breeding seahorses is correlated with parental body size and size of pouch. This is logical, as larger males, with larger pouches, are not only able to accept more eggs from females, but also have the capacity to nurture them. During early development, the seahorse embryos attach themselves to depressions in the inner surface of the brood pouch and, as mentioned above, establish close physiological and structural interactions that resemble the mammalian placenta. Simple inspection of the pouch inner surface shows that some of these depressions are rather shallow, while others are deep, and perhaps capable of providing higher-quality functional interactions with embryos.
The seahorse pouch is now known to be much more than a simple incubator. A recent study[5] aimed at determining gene expression changes during Hippocampus abdominalis pregnancy revealed a number of similarities between seahorse and mammalian pregnancy. The pouch transcriptome becomes highly modified during pregnancy, with upregulation of genes involved in, for example, tissue remodelling, bacterial suppression, lipid and ion transport, and immune regulation. Apolipoprotein A, a lipid transport protein, and transferrin/transferrin receptor were especially significant in this regard, as they are both significantly upregulated during human pregnancy. Also of interest is oviductin (also known as oviductal glycoprotein 1), a member of the mucin family, which was highly upregulated during pregnancy. This protein is familiar to reproductive biologists who work on sperm transport and storage in mammals, as it is upregulated within the oviduct, or fallopian tube, before ovulation and has a role in fertilisation.
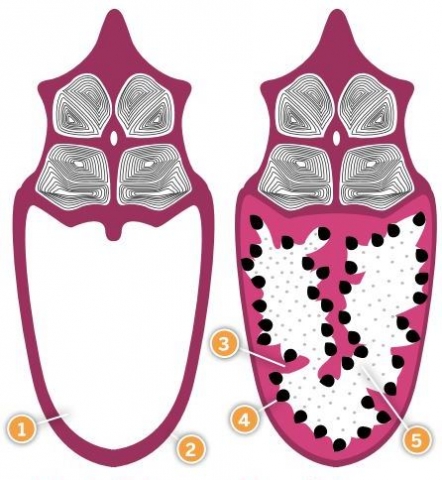
We still don't know whether, and how, the nutritional status of male and female parents affects the development and survival of their offspring. It could be argued that if seahorse embryos are well provisioned via the yolk sac, their development would depend mainly on the nutritional status of females. But if, as we suspect, pouch function is sophisticated, the male's health may have an impact on the physiological fitness and survival of the offspring. In mammals, it is increasingly clear that there are complex relationships between offspring fitness and maternal body condition, both before and after conception.
There is evidence in humans and other species to support the view that poor periconception diets predispose offspring to developing diseases such as diabetes and hypertension later in life.
In a 2014 study[6] we therefore investigated the hypothesis that the Hippocampus reidi's paternal pouch environment around conception might be similarly influential in terms of determining juvenile growth and survival. Adult male and female seahorses were fed separately with two types of diet: the first a high-quality 'wild' (W) diet, the second a 'commercial' (C) diet, widely used by commercial aquaria. (The main difference between the diets was that the commercial diet was slightly less rich in the essential fatty acids linolenic acid and arachidonic acid.)
After being fed on the different diets, individuals were allowed to breed freely over a period of five months without any further dietary changes. This experimental design produced four gender/diet breeding combinations: MW FW, MW FC, MC FW and MC FC, where male and female are represented as M or F and W and C represent their diet.
The mean brood size was highest in treatments that involved females fed a wild-caught diet (FW) and lowest where both males and females were fed the commercial diet. The overall importance of female diet was clear, with all offspring of the commercial diet females being significantly smaller, an effect that could not be rescued by breeding with wild-diet males.
However, offspring survival after 15 days was significantly higher in the MW/FW treatment (39%) compared with the MC/FW treatment (12.9%).
This outcome indicates that pouch function and some aspects of offspring physiology must have been negatively affected by feeding the lower-quality diet to males.
Interestingly, the MC FW combination produced juveniles whose body length was significantly longer than those in other treatments – they were found to have particularly long tails. This indicates that giving the poorer-quality diet to males tended to induce a form of phenotypic distortion, whereby body parts were growing at different rates and producing detrimental effects on fitness.
A similar effect was detected in juveniles produced from the MW FC treatment, which showed distortion of the snout length/head length ratio (see image, p12, bottom). This distortion would be especially important in terms of juvenile survival because it would affect their ability to generate suction, an important aspect of feeding given the species' lack of a jaw.
Seahorses, pipefishes and their exotic cousins the sea dragons belong to a large group of species known as the Syngnathidae – the systematic name referring to the fused jaws they all possess. Seahorses are widely distributed throughout tropical, subtropical and temperate regions of the world, and can be found as far north as Canada and even in the river Thames.
Their unusual shape and behaviour has led to them being over-exploited commercially by the aquarium trade and as ornamental curios[8], and unfortunately there is a massive demand for them in the preparation of traditional oriental medicine. Recent estimates suggest that somewhere between 64 million and 150 million seahorses are killed annually to service these markets.
The exact number of seahorse species is uncertain, but the International Union for Nature Conservation's Red List of threatened species provides data on 44 species. Two of these (Hippocampus capensis and Hippocampus whitei) are listed as endangered, 11 are seen as vulnerable and 17 species are classified as data deficient.
It is important to realise that our simple experimental treatments would never occur in nature, and so it seems that one important reason we detected various effects on growth and development was due to providing mismatched diets to the male and female parents.
Mismatched diets crop up frequently in the literature about developmental programming[7] – it is increasingly accepted that if early mammalian embryos experience poor nutritional conditions in utero, they become programmed to cope with poor nutrition after birth. (If they subsequently experience much richer diets than expected, they are likely to develop diseases such as diabetes and hypertension in later life).
In the case of seahorses, we speculate that when eggs from well-fed females are fertilised by sperm from less well-fed male partners and they have to develop within the pouches of these males, the resultant mismatch produces poor developmental outcomes.
In this respect, the seahorse presents itself as an unusually useful experimental model for investigating foetal programming effects. In mammals, it would be impossible to manipulate egg quality independently from uterine function, while in principle in seahorses this type of manipulation is perfectly feasible.
Francisco Otero-Ferrer is a researcher at the Grupo en Biodiversidad y Conservación, IU-ECOAQUA, at the University of Las Palmas Gran Canaria, Spain. William V Holt MRSB is a professor of reproductive science working at the Academic Unit of Reproductive and Developmental Medicine at the University of Sheffield.
1) Stockley, P. et al. Sperm competition in fishes: the evolution of testis size and ejaculate characteristics. Am. Nat. 149, 933–954 (1997).
2) Dzyuba, B. B. et al. Variable sperm size and motility activation in the pipefish Syngnathus abaster: adaptations to paternal care or environmental plasticity? Reprod. Fert. Dev. 20, 474–482 (2008).
3) Van Look, K. J. W. et al. Dimorphic sperm and the unlikely route to fertilisation in the yellow seahorse. J. Exp. Biol. 210, 432–437 (2007).
4) Watanabe, S. et al. Male internal fertilization and introsperm-like sperm of the seaweed pipefish (Syngnathus schlegeli). Zoological Science 17, 759–767 (2000).
5) Whittington, C. M. et al. Seahorse brood pouch transcriptome reveals common genes associated with vertebrate pregnancy. Mol. Biol. Evol. 32, 3114–3131 (2015).
6) Otero-Ferrer, F. et al. Embryonic developmental plasticity in the long-snouted seahorse (Hippocampus reidi, Ginsburg 1933) in relation to parental preconception diet. Reprod. Fert. Dev. 28, 1020–1028 (2014).
7) Bateson, P. et al. The biology of developmental plasticity and the predictive adaptive response hypothesis. J. Physiol. 592, 2357–2368 (2014).