The plastic eaters
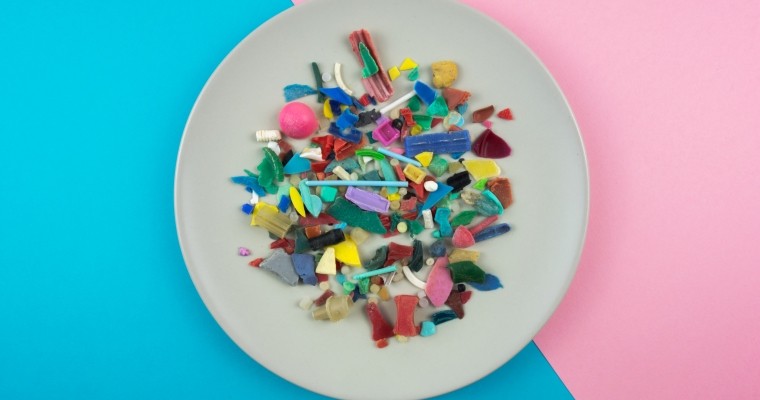
Evidence that some microbes have evolved the ability to metabolise plastic waste has raised hopes they could help clear up our oceans and landfill sites. James Romero explores the difficult realities of this approach
Microbes are Earth’s great recyclers. Anything that decays does so thanks to the unending appetite and diverse palate of the planet’s microorganisms. In the last decade, we have found evidence that a new item has been added to the microbial menu, one unlike anything evolution has cooked up before. That item is plastic, a substance that we have added to virtually every environment on Earth. Now scientists are asking whether the discovery of plastic-munching bacteria could help clean up our oceans and empty our landfill sites.
Plastic is the term given to a range of synthetic or semi-synthetic materials formed from carbon-rich polymers. Altering the chemical backbone or side chains of these polymers gives rise to materials with a variety of useful properties. Plastics’ stability and durability provided a convenient way to protect the products of our increasingly consumerist society from the outside world. Unfortunately, the need to protect the outside world from them wasn’t such a major consideration.
A 2017 study by the University of California’s Roland Geyer[1] found that of the approximately 9.2 billion tonnes of plastic produced since 1950, seven billion tonnes became waste. Much of it has ended up in our oceans, where almost 200 trillion plastic particles can now be found, according to recent estimates[2].
Whether in landfill or in the sea, the risks plastic poses to life are varied – from the threat of physical strangulation or choking to the dangers of their toxic degradation products leaching out into the environment. There is also evidence of plastic particles helping to facilitate bacterial infection[3], as well as breaking down into microplastic particles so fine that they can pass into our bloodstream[4].
A penchant for PET
Against this backdrop there was understandable excitement around the discovery of plastic metabolising species. In 2016 a new species, Ideonella sakaiensis, recovered from a bottle-recycling facility in Sakai, Japan, hit the headlines for its ability to almost completely break down polyethylene terephthalate (PET)[5], one of the world’s most used plastics found in drinks bottles, clothing, packaging and carpeting.
A few years after the discovery of the original Ideonella sakaiensis microbe in Japan, scientists at the University of Portsmouth inadvertently tweaked the microbe’s key plastic-degrading enzyme, resulting in it performing 20% better at breaking down PET[6].
Since then other types of plastic have proved to be a microbial food source. In 2020 a US and UK collaboration discovered Pseudomonas capeferrum feeding on polyurethane[7], a plastic used in sports shoes, nappies, kitchen sponges and foam insulation. This was a particularly surprising discovery given the breakdown of polyurethane is known to release chemicals that are toxic to most bacteria.
In both of these cases the plastic degradation was aided by cocktails of enzymes secreted by the microbes. These generally contain a subset of enzymes that act directly on the solid polymers and complementary enzymes that process intermediate states, eventually producing carbon-based monomers like glucose. These monomers can then be assimilated by the microbe to build cell components such as DNA and lipids, or be used for energy within oxidation or reduction reactions.
At this point it might be tempting to think nature can bail us out of our plastic problem, as evidence of microbial life hungry for our plastic waste emerges. However, most analysis of decomposition rates in the real world, rather than the laboratory, suggest that even with the right microbes present, plastics such as PET will be in the environment for centuries. And it will be much longer for other common varieties such as polyethylenes and polystyrenes.
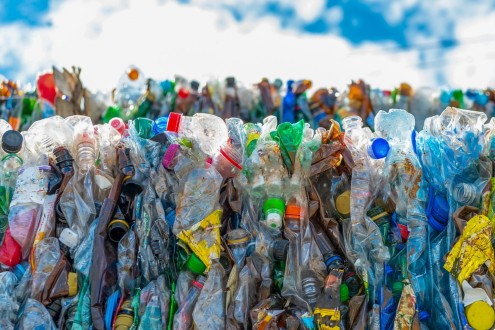
Controlled approach
There are opportunities to use microbes in the right setting - but it is unlikely to involve spraying them onto the floating rubbish heaps building up in our oceans. Introducing engineered microbes into the natural environment poses many ethical questions and the naturally occurring strains discovered so far mostly only work on PET-type plastics, which don’t tend to stay near the surface long enough.
But microbial breakdown might be possible in controlled, self-contained environments.
“I can imagine that in wastewater treatment plants or other locations where they find a lot of nano and microplastics, it might be a possibility to clean it [with microbial enzymes],” says Bruce Lichtenstein, a protein engineer interested in plastic degradation at the University of Portsmouth’s Centre for Enzyme Innovation. However, he is clear that microbes alone are not the answer. “I think that we have some responsibility as stewards of the Earth to make sure that nature is not the fallback here,” he says.
Another opportunity for microbial solutions requires us to look beyond the full breakdown of the plastic and focus instead on the final output. One commercial leader in the field of plastic breakdown, France’s Carbios, is working on an optimised PET hydrolase enzyme that can break down waste PET to the extent that it can be reformed into ‘biologically recycled PET’ as part of a circular economy[8].
Novel Products
Microbes are not just degraders – they are recyclers and green manufacturers that can produce all sorts of materials with the right conditions and feedstock. So while plastic polymers have few natural equivalents, the monomers they break down into do. For example, styrene, the monomer of polystyrene, is naturally produced by plants during their decomposition. As a result, there are many microbes out there that will happily metabolise degraded plastic monomers and various teams are starting to demonstrate how such a metabolic process can create new products.
In one neat example, a team from the University of Edinburgh used engineered biological enzymes to turn TPA, a degradation product of PET plastics, into vanilla flavouring[9]. “You can take a bottle of vanilla Coke and turn it into the flavouring that was in that Coke bottle,” says Lichtenstein.
Others are looking at using microbes to turn waste plastics into new bioplastics that biodegrade more easily. This is the aim of University College Dublin’s Kevin O’Connor, director of the circular bioeconomy research collaboration BiOrbic. His team’s approach uses enzymatic degradation to break down PET, then microbial biosynthesis with engineered Pseudomonas umsongensis to convert the breakdown products into the monomers that can form two types of bioplastic: bio-polyamide urethane and polyhydroxyalkanoate.
With plastic production accounting for 3.4% of global carbon emissions[10], it is a plastic clean-up approach that could help fight climate change by reducing new plastic production. Currently, biodegradable plastics make up just 1% of the total plastics produced around the world[11], but it is a growing market, with double-digit growth per year. One of the leading bioplastic products is polylactic acid, used to make foams, fibres and even packets of Lays crisps. (Sadly, the latter was withdrawn due to customers complaining the greener packaging was too noisy.)

Degradation difficulties
There are bigger barriers to overcome than noise complaints. The crude oil required to make new plastic is still cheap, often due to government subsidies, and virgin plastic still makes more economic sense than producing it from recycled waste.
More fundamental for microbial approaches is the issue of degradation rates. Maaike Goudriaan, whose research at the Royal Netherlands Institute for Sea Research has been quantifying microbial metabolism rates of plastic in different environments, says breakdown rates of around 1% per year pose difficult questions for how this approach can have any real impact at scale. “I don’t see it happening immediately,” she says, while acknowledging rates could be increased by genetic engineering in the future.
“I think we will make more advances there [in genetic engineering],” agrees O’Connor. “But right now, if you want to degrade a tonne of plastic, the microbial enzymes are still too slow.” Even his second-phase microbial manufacturing line in recycled biodegradable plastics is some way off where it needs to be for commercialisation. “We’re still only at the kind of kilogram scale [in terms of biodegradable plastic produced] and we need to be at the multi-thousand tonne scale.”
Another issue is the range of plastics in use around the world. “If we’re being honest … the number of plastics that have actually been properly decomposed by enzymes or biochemically is not that high,” admits Lichtenstein. Microbial enzymes may be showing promise in substance such as PET, but the bonds in polyethylene or polystyrene are so strong that they are likely to need an industrial chemical approach to be broken down at scale.
A rethink from the ground up
And this brings us back to a fundamental point with regards to our plastic problem: the majority of plastics in use today are still designed without any end-of-life plan. We can focus on the clean-up, where encouraging lab results suggest microbial degraders and recyclers can play a part. But what we really need is a rethink on plastic as a whole from further upstream.
That means “redesigning plastics from the ground up”, says Lichtenstein, so the end products are designed with microbial solutions in mind. At the same time, we need better processes for collecting and preparing plastic waste to ensure high-quality monomers can be produced more affordably and on an industrial scale.
And, finally, we need new business practices and government incentives that make upcycled or recycled biodegradable plastic competitive with new fossil-fuel-derived ones.
Only when all that is achieved can microbes build on their 75 years of adaptation to our modern plastic society and maintain their reputation as the greatest recycling force in Earth’s history.
James Romero is a UK-based science writer covering new research stories across the fields of geology, archaeology, astronomy, cosmology and planetary science. Outside of his science writing he also organises science festivals and exhibitions in his home city of London.
1) Geyer, R. et al. Production, use, and fate of all plastics ever made. Sci. Adv. 3(7) (2017).
2) Eriksen, M. et al. A growing plastic smog, now estimated to be over 170 trillion plastic particles afloat in the world’s oceans – Urgent solutions required. PLoS ONE (2023).
3) Kirstein, I. V. et al. Dangerous hitchhikers? Evidence for potentialy pathogenic Vibrio spp. on microplastic particles. Mar. Environ. Res. 120, 1–8 (2016).
4) Leslie, H. A. et al. Discovery and quantification of plastic particle pollution in human blood. Environ. Int. 163, 107199 (2022).
5) Yoshida, S. et al. A bacterium that degrades and assimilates poly(ethylene terephthalate). Science 351(6278), 1196–1199 (2016).
6) Austin, H. P. et al. Characterisation and engineering of a plastic-degrading aromatic polyesterase. PNAS 115(19), E4350–E4357 (2018).
7) Puiggené, Ò. et al. Extracellular degradation of a polyurethane oligomer involving outer membrane vesicles and further insights on the degradation of 2,4-diaminotoluene in Pseudomonas capeferrum TDA1. Sci. Rep. 12, 2666 (2022).
8) www.carbios.com/en/enzymatic-recycling
9) Sadler, J. C. & Wallace, S. Microbial synthesis of vanillin from waste poly(ethylene terephthalate). Green Chem. 23, 4665 (2021).
10) Plastic leakage and greenhouse gas emissions are increasing. OECD.
11) World plastics production 2021. Plastics Europe, 2022.